The
Warsaw cyclotron facility with a horizontal beam for radiobiological
studies.
Joanna Czub (1), Tomasz Adamus (2), Dariusz Banaś (1), Janusz Braziewicz
(1), Jarosław Choiński (5), Jan Dyczewski (2),
Marian Jaskóla (4), Andrzej Korman (4),
Zygmunt Szefliński (2), Andrzej Wójcik (3),
(1) Institute of Physics, Świętokrzyska Academy, Kielce, Poland
(2) Nuclear Physics Division, Institute of Experimental Physics, Warsaw
University,
(3) Institute of Biology, Świętokrzyska Academy, Kielce, and Institute of
Nuclear Chemistry and Technology, Warsaw, Poland
(4) The Andrzej Soltan Institute for Nuclear Studies, Otwock - Świerk,
Poland
(5) Heavy Ion Laboratory, Warsaw, Poland
The
understanding of radiobiological effects of charged heavy particles
are of fundamental importance both for radiation protection and
radiotherapy, where the number of patients treated with heavy ions is
increasing [1,2]. The physical environment of the sample is the major
problem in biological studies of living cells under irradiation by
the uniform beam of heavy ions. The interaction of densely ionizing
particles with DNA in the cell nucleus yields important information
to the models describing both the track structure, the DNA-geometry
as well as the effect of radiation on the cell. The knowledge of
radiation-induced DNA-breaks allows, for instance, to estimate the
risks from radiation exposure at low doses (e.g. during long-manned
space flights). The technical realization of a particle irradiation
facility with a horizontal beam-line at the HIL is now tested.
In
the experiment at the Warsaw Cyclotron horizontal beams of the 100-60
MeV 12C ions are extracted to the atmosphere from the
vacuum tube through an exit window made from the Havar foil 2.5
mg/cm2 thick with a size of 13 x 13 mm.
The Au scattering foils of different thickness 20 - 60 mg/cm2
are used to produce a uniformly distributed beam directed to exit
window placed at 00 and to the beam monitor located at
150. A sketch of the horizontal beam line of the HIL is
shown in Fig.1.The uniform spatial distribution of the beam intensity
at the exit window is assured by the small-angle multiple Rutherford
scattering events inside the foil. The cell containers fastened to an
X-Y sliding table (Fig. 2) is moved along the X and Y axes in order
to ensure a uniform dose distribution at the irradiated target.
Horizontal beam line
With the
Warsaw cyclotron almost all types of ions with high linear energy
transfer (LET) can be accelerated. The beams are transported by a
horizontal line with conventional beam tuning components including a
fast mechanical beam shutter, which can be activated by the
irradiation control system. The beams leave the vacuum tube through
an exit window with a size 13*13mm sealed by a 2.5 mg/cm2
thick Havar foil.
The
thickness of all materials passed by the beams is controlled to
assure the desirable energy, i.e. in the region of the Bragg peak, at
our radiobiological experiments. Finally, the energy loss in the
irradiated cells can be varied from ~4 keV/μm
to ~50 keV/μm.
Fig. 1. A Sketch of the set up for cell
irradiation at Warsaw cyclotron
Beam spreading
To achieve
a homogeneous radiation field outside the exit window the beam is
spread out using Au foils of appropriate thickness. For different
beam energies and types of ions a suitable Au-foil can be rotated
into the beam by a foil-changer wheel. Test measurements and
Monte-Carlo calculations of the beam widening show that for a 100 MeV
12C ions a Au foil with a thickness of 40 mg/cm2
is adequate to assure the beam homogeneity better than 5% across the
exit window 13*13 mm (see Fig. 3).
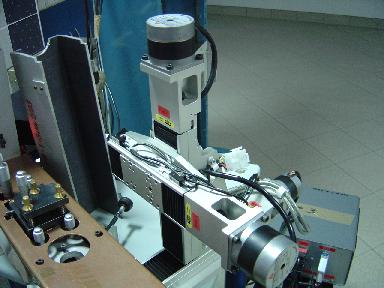 | The cell
containers fastened to an X-Y sliding table (Fig. 2) is moved along
the X and Y axes in order to ensure a uniform dose distribution at
the irradiated target at a standard petri dish with the diameter 60
mm (see Fig 1). |
Fig 2. The X-Y sliding table |
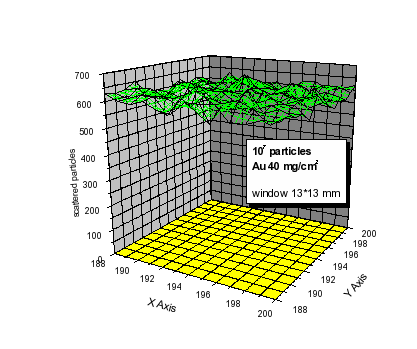
The Monte
Carlo method is adopted to simulate the beam intensity at real
experimental conditions, i.e. passing through Au-, Havar-, air- and
Mylar-foils. The beam homogeneity spreaded on the irradiated
biological area window is better than 5% (see Fig. 3).
Fig.3 Two-dimensional plot of the
number of particles at the irradiated target.
Beam monitoring
The
intensity of the beam scattered at the gold scattering foil is
measured by a barrier silicon detector placed at 150. To
reduce the count rate, a collimator with 2mm diameter is placed in
front of the detector. The detector is placed at a distance of 125mm
from the center of the scattering chamber, where the scattering foil
is mounted. Appropriate Monte-Carlo multiscattering simulations were
performed to correlate the intensity of the scattered particles at
the RBS detector and the spatial distribution at the target position.
The intensity and the distribution of the scattered beam is measured
at the petri dish position in off-line measured by x-ray films.
Fig. 4. The result of irradiation of
the X-ray film irradiated with doses 4.7 Gy (left),
9.5 Gy (center) and 18.9 Gy (right).
Simultaneously,
the data acquisition
system is registering time dependence of the beam intensity as well
as the energy spectrum of the scattered beam. A selected number of
counted particles initiates the programmed shift of the sliding table
with the sample.
Fig. 5. The scheme of the control system of the irradiation.
In
order to perform uniform irradiation of biological samples, which are
spread over 6 cm in diameter, the moving sample is scanned by the
beam passively smeared by the scattering foil. The required flux of
the particles 107/ s of 12C at incident energy
100 MeV corresponds to the dose rate 10 Gy/min. The scanning in the
horizontal and vertical direction is performed to obtain a
homogeneity of the beam in the order of <5%. The dose
determination based on the counting of ions scattered at 150
leads to the determination of number of particles reaching the
sample. To verify the dose a set of thermoluminescence detectors, as
well as an X-ray film are irradiated. The results of irradiation of
the X-ray film irradiated with doses 0.25 Gy, 0.5 Gy, and 1.0 Gy
respectively are shown in Fig. 5.
First experiment
V79 Chinese
hamster fibroblasts were grown on thin foils for 24 hours,
transported to the Heavy Ion Laboratory on ice and irradiated for
different time periods in order to determine the optimal doses and
exposure times that yield enough mitotic cells for scoring of
chromosomal aberrations. Following irradiation the cells were
transported on ice back to the laboratory where they were incubated
at 37oC for 16 hours. Colcemid was added for the last 3
hours in order to block cells in mitosis. Cells were harvested as
described elsewhere and aberrations were scored under a light
microscope [3]. An example of a cell
with chromosomal aberrations is shown in figure 6. A good yield of
mitotic cells was observed after exposure times of 10-15 minutes and
doses of 0.5-1 Gy. These exposure conditions will be used in future
experiments.
Fig 6. An example of a
mitotic cell with chromosomal aberrations.
References:
[1] Wada S. et al.
Detection of DNA damage induced by heavy ion irradiation
in the
individual cells with comet assay.
Nucl. Instr. and Meth. in Phys. Research B206(2003)553-556.
[2] Kraft G. Radiobiological
Effects of Highly Charged Ions. in: The
Physics of Highly and
Multiply Charged Ions,
Academic Publisher (2002)
[3] Wojcik A. et al. Rad51C-deficient CL-V4B
cells exhibit normal levels of mitomycin C-induced SCEs but reduced
levels of UVC-induced SCEs
Biochem. Biophys. Res. Commun. 326(2005):805-10.
|